Effective c++
item1 把c++看成语言的集合
C++可以看成由几个模块组成:
- C:C++是以C为基础的,指针、数组、数据类型等都是来自于C
- OOC(Object-Oriented C++):类、继承、多态、虚函数等
- Template:泛型模块
- STL
这样区分的目的是:不同的模块有不同的编程策略
- C模块中,一般用值传递
- OOC,常量引用传递
- STL,也是使用值传递
item2 用const、enum、inline代替#define
尽量以编译器代替预处理器
方便在编译出错的时候找到错误位置,因为编译器对变量报错是根据符号表来的,宏至少简单的文本替换,出错的时候不容易定位。
- 用const用来声明数值常量、常量指针、类中的常量需要加上static,从而保证不会产生多个拷贝
- enum hack:enum的行为像#define,比如不能获得地址,不会引来非必要的内存分配
- 使用inline代替宏:对于简单的语句封装成函数不划算,但是用宏可能会带来一些不可预料的错误,尽量写templet inline函数。
item3 多用const
const的使用范围
- 可以在class外部修饰global或namesspace作用域中的常量
- 可以修饰被声明为static的对象
- 也可以修饰class内部的static和non-static成员变量
- 指针自身,指针所指也可以是const
const的语法规则
const int* ptr
被指的是常量,也就是指向的内存不能修改
int* const ptr
指针本身是常量,指针指向的位置不能修改
const int* ptr
和int const* ptr
,意义相同
STL迭代器的const
- 对于STL的迭代器,如果希望迭代器所指的东西不可变动,需要使用的是const_iterator,如
std::vector<int>::const_iterator const_itr = vec.begin()
- 如果迭代器本身不可变动,即不可指向别的地方,直接用const修饰即可
const在声明函数使用
const可以修饰函数的返回值、函数参数、函数自身
const Rational operator* (const Rational& lhs, const Rational& rhs);
const成员函数
指明该函数不会修改类的任何成员数据的值
bitwise constness和logical constness
1 2 3 4 5 6 7 8 9
| class BigArray { vector<int> v; int accessCounter; public: int getItem(int index) const { accessCounter++; return v[index]; } };
|
这个是编译不过的。我们希望的是getItem不会修改核心成员,而不考虑非核心成员,是logical constness。
编译器只认bitwise constness,为了解决这个问题,用mutable修饰accessCounter:mutable int accessCounter;
。
const和non-const成员函数的重复问题
为了避免代码重复,使用non-const函数调用const函数。
关于const约束的变量
从变量的名称开始,顺时针移动到下一个指针或者类型,直到表达式结束
或者是从右到左的语法解码,后面的修饰前面的
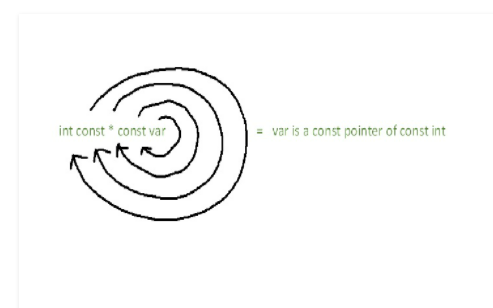
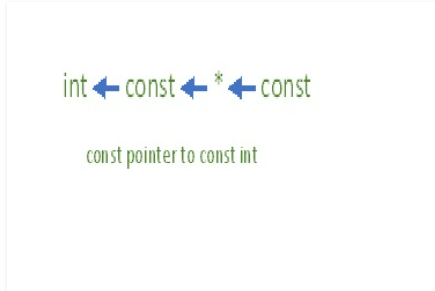
*
读作pointer to
int const *p
:p是一个指针,指向一个const int,也就是说p本身指向的位置是可以修改的,但是指向的内存不能修改
int * const p
:p是一个const指针,指向int,就是说这个指针指向的位置不能修改,但是允许修改存储在地址的值
const int* cont p
:p是一个const 指针,指向一个const int。p既不能修改指向的位置,也不能修改里面的值
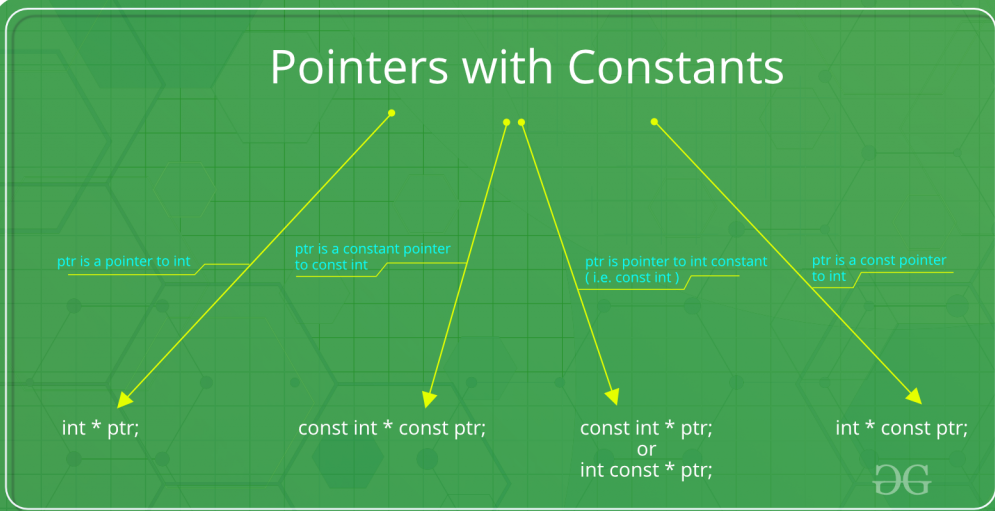
item 4确定对象被使用之前已经被初始化
永远在对象使用之前初始化
对于基本数据类型,手工完成。对于类,写构造函数,使得对象的每一个成员都被初始化。
主要:赋值和初始化不同
赋值与初始化
语法
虚函数
http://www.noobyard.com/article/p-yfcqfueo-su.html
为什么要有虚函数:为了实现动态绑定,即在运行时决定调用哪个函数。举个例子:person为基类,派生出student 和 teacher子类,现在学校大门口有一个队列,存放了一系列person指针,每次pop,通过ptr调用函数“出校”,区别是学生需要扫码,而教师不需要,但是编译器在编译程序的时候并不知道要调用哪个出校函数,这取决与运行时队列pop出来的到底是student还是teacher,也就是动态绑定的含义,通过对象的虚表指针调用,因此也可以理解为:虚函数是将函数绑定到特定类的一种手段。
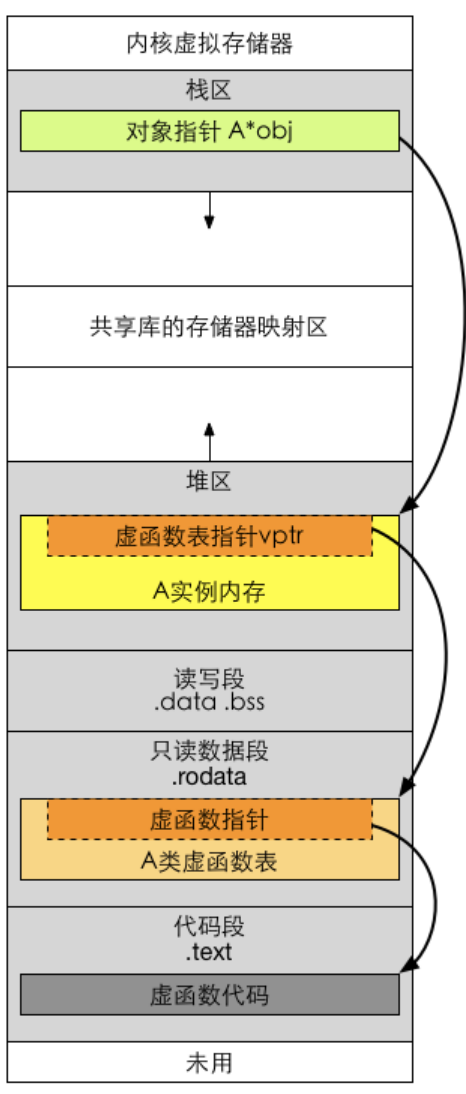
static关键字
https://zhuanlan.zhihu.com/p/37439983
static出现在两种场景:面向对象和面向过程
静态成员变量
为什么要用static成员变量?每个对象有用相同的某项属性,就用static,比如student类,包含static int num 学生总人数,这是每个学生实例公用的。
static成员变量分配在数据段
跟全局对象相比:静态成员变量的命名空间在类中,不会与全局命名空间的命名产生冲突。static可以加入访问控制,比如private。
静态成员函数
为什么用static成员函数?类似的,static成员函数为类服务,而不是为对象服务,因此static成员函数也没有this指针,因此也仅可以访问静态成员函数和静态成员变量
静态成员函数不能访问非静态成员函数和变量
反过来非静态成员函数可以任意访问静态成员函数和变量
静态全局变量
静态局部变量
静态函数
static函数仅在声明他的函数中可见,不能被其他文件使用
为什么用?不同文件中可以定义相同名字的函数,不会发生冲突。
RAII
modern C++
完美转发std::forward
RAII和智能指针
RAII
RAII是c++特有的资源管理方式。把依赖栈和析构函数,对所有资源进行管理(包括堆内存,下面有一个例子)。栈上的指针在释放的时候会调用析构函数,在析构函数里释放堆内存。
例子:
create_shape在堆上new了对象,返回对象的指针,如何让这块内存不会泄露?
把这个函数的返回值(对象的指针),包裹到一个局部对象中,该局部对象的析构函数中delete掉这个指针。
1 2 3 4 5 6 7 8 9 10 11 12 13 14 15 16 17 18 19 20 21 22
| class shape_wrapper { public: explicit shape_wrapper( shape* ptr = nullptr) : ptr_(ptr) {} ~shape_wrapper() { delete ptr_; } shape* get() const { return ptr_; } private: shape* ptr_; };
void foo() { … shape_wrapper ptr_wrapper( create_shape(…)); … }
|
经典RAII的例子:
1 2 3 4 5 6 7 8
| std::mutex mtx;
void some_func() { std::lock_guard<std::mutex> guard(mtx); }
|
而不写成:
1 2 3 4 5 6 7 8 9 10 11
| std::mutex mtx;
void some_func() { mtx.lock(); mtx.unlock(); }
|
智能指针实现
从刚刚shape_wrapper类改造成模板类,就是一个简单的智能指针:
1 2 3 4 5 6 7 8 9 10 11 12 13 14
| template <typename T> class smart_ptr { public: explicit smart_ptr(T* ptr = nullptr) : ptr_(ptr) {} ~smart_ptr() { delete ptr_; } T* get() const { return ptr_; } private: T* ptr_; };
|
但是这样跟指针的行为还有点差异,比如不能用*解引用,不能用->访问成员,不能用在bool表达式里,因此添加:
1 2 3 4 5 6 7 8 9
| template <typename T> class smart_ptr { public: … T& operator*() const { return *ptr_; } T* operator->() const { return ptr_; } operator bool() const { return ptr_; } }
|
然后对于拷贝构造呢?smart_ptr<shape> ptr2(ptr1)
应该怎么表现?
转移指针所有权,这就是auto_ptr的实现了,现在已经废除,因为这样设计的smart_ptr一旦进行了赋值或拷贝,就不在拥有这个对象了。
1 2 3 4 5 6 7 8 9 10 11 12 13 14 15 16 17 18 19 20 21 22 23 24 25 26 27 28
| template <typename T> class smart_ptr { … smart_ptr(smart_ptr& other) { ptr_ = other.release(); } smart_ptr& operator=(smart_ptr& rhs) { smart_ptr(rhs).swap(*this); return *this; } … T* release() { T* ptr = ptr_; ptr_ = nullptr; return ptr; } void swap(smart_ptr& rhs) { using std::swap; swap(ptr_, rhs.ptr_); } … };
|
再改下,就是unique_ptr:
1 2 3 4 5 6 7 8 9 10 11 12 13 14 15
| template <typename T> class smart_ptr { … smart_ptr(smart_ptr&& other) { ptr_ = other.release(); } smart_ptr& operator=(smart_ptr rhs) { rhs.swap(*this); return *this; } … };
|
现在添加引用计数,形成shared_ptr:
1 2 3 4 5 6 7 8 9 10 11 12 13 14 15 16 17 18 19 20 21 22 23 24 25 26 27 28 29 30 31 32 33 34 35 36 37 38 39 40 41 42 43 44 45 46 47 48 49 50 51 52 53 54 55 56 57 58 59 60 61 62 63 64 65 66 67 68 69 70 71 72 73 74 75 76 77 78 79 80 81 82 83 84 85 86 87 88 89 90 91 92 93 94 95 96 97 98 99 100 101 102 103 104 105 106 107 108 109 110 111 112 113 114 115 116 117 118 119 120 121 122 123 124 125 126 127 128 129 130 131 132 133 134 135 136 137 138 139 140 141 142 143 144 145 146 147 148 149 150 151 152 153 154 155 156 157 158 159 160 161 162 163 164 165 166 167 168 169 170 171 172 173
| #include <utility>
class shared_count { public: shared_count() noexcept : count_(1) {} void add_count() noexcept { ++count_; } long reduce_count() noexcept { return --count_; } long get_count() const noexcept { return count_; }
private: long count_; };
template <typename T> class smart_ptr { public: template <typename U> friend class smart_ptr;
explicit smart_ptr(T* ptr = nullptr) : ptr_(ptr) { if (ptr) { shared_count_ = new shared_count(); } } ~smart_ptr() { if (ptr_ && !shared_count_ ->reduce_count()) { delete ptr_; delete shared_count_; } }
smart_ptr(const smart_ptr& other) { ptr_ = other.ptr_; if (ptr_) { other.shared_count_ ->add_count(); shared_count_ = other.shared_count_; } } template <typename U> smart_ptr(const smart_ptr<U>& other) noexcept { ptr_ = other.ptr_; if (ptr_) { other.shared_count_->add_count(); shared_count_ = other.shared_count_; } } template <typename U> smart_ptr(smart_ptr<U>&& other) noexcept { ptr_ = other.ptr_; if (ptr_) { shared_count_ = other.shared_count_; other.ptr_ = nullptr; } } template <typename U> smart_ptr(const smart_ptr<U>& other, T* ptr) noexcept { ptr_ = ptr; if (ptr_) { other.shared_count_ ->add_count(); shared_count_ = other.shared_count_; } } smart_ptr& operator=(smart_ptr rhs) noexcept { rhs.swap(*this); return *this; }
T* get() const noexcept { return ptr_; } long use_count() const noexcept { if (ptr_) { return shared_count_ ->get_count(); } else { return 0; } } void swap(smart_ptr& rhs) noexcept { using std::swap; swap(ptr_, rhs.ptr_); swap(shared_count_, rhs.shared_count_); }
T& operator*() const noexcept { return *ptr_; } T* operator->() const noexcept { return ptr_; } operator bool() const noexcept { return ptr_; }
private: T* ptr_; shared_count* shared_count_; };
template <typename T> void swap(smart_ptr<T>& lhs, smart_ptr<T>& rhs) noexcept { lhs.swap(rhs); }
template <typename T, typename U> smart_ptr<T> static_pointer_cast( const smart_ptr<U>& other) noexcept { T* ptr = static_cast<T*>(other.get()); return smart_ptr<T>(other, ptr); }
template <typename T, typename U> smart_ptr<T> reinterpret_pointer_cast( const smart_ptr<U>& other) noexcept { T* ptr = reinterpret_cast<T*>(other.get()); return smart_ptr<T>(other, ptr); }
template <typename T, typename U> smart_ptr<T> const_pointer_cast( const smart_ptr<U>& other) noexcept { T* ptr = const_cast<T*>(other.get()); return smart_ptr<T>(other, ptr); }
template <typename T, typename U> smart_ptr<T> dynamic_pointer_cast( const smart_ptr<U>& other) noexcept { T* ptr = dynamic_cast<T*>(other.get()); return smart_ptr<T>(other, ptr); }
|
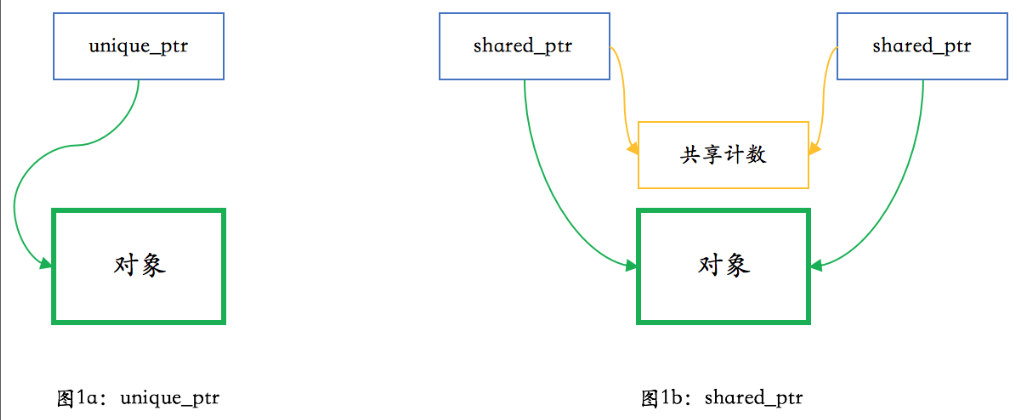
问题
- shared_ptr怎么实现的
- shared_ptr是否线程安全
- 分场景,同一个shared_ptr被多个线程写,不是线程安全的。多线程读是安全的,根据同一个智能指针创建新的智能指针(增加引用计数)也是安全的。(不懂)
atomic
c++11引入atomic模板,可以应用到任何类型上,但实现不一样
对于整型量和指针等简单类型,通常结果是无锁的原子对象;
而对于另外一些类型,比如 64 位机器上大小不是 1、2、4、8(有些平台 / 编译器也支持对更大的数据进行无锁原子操作)的类型,编译器会自动为这些原子对象的操作加上锁。
编译器提供了一个原子对象的成员函数 is_lock_free,可以检查这个原子对象上的操作是否是无锁的。
问题
std::function
std::function是一个可调用对象的包装器
可调用对象可以是:函数指针、一个有operator成员函数的类对象、可以被转换成函数指针的类对象、类成员函数指针。
比如:
1 2 3 4 5 6 7 8 9 10 11 12
| int add(int a, int b){return a+b;}
auto mod = [](int a, int b){ return a % b;}
struct divide{ int operator()(int denominator, int divisor){ return denominator/divisor; } };
|
虽然他们类型不同,但是调用形式是相同的,调用形式就是参数和返回值,也就是function初始化时候的模板参数,于是就可以这样写:
1 2 3
| std::function<int(int ,int)> a = add; std::function<int(int ,int)> b = mod ; std::function<int(int ,int)> c = divide();
|
用来取代函数指针,特别适合作为回调函数使用。
std::bind
把可调用实体的某些传入参数,绑定到已有的变量,返回一个std::function
1 2 3 4 5 6 7 8 9 10 11 12 13
| struct Foo { void print_sum(int n1, int n2) { std::cout << n1+n2 << '\n'; } int data = 10; }; int main() { Foo foo; auto f = std::bind(&Foo::print_sum, &foo, 95, std::placeholders::_1); f(5); }
|
通过std::bind和std::function配合使用,所有的可调用对象均有了统一的操作方法。
auto和decltype
auto 的推导用在初始化时候,但目前的c++标准不允许类成员变量使用auto。
auto总是推导出值类型,不可能是引用。
auto可以加上const、volatile、*、& 这样的类型修饰符,得到新的类型。
STL
参考